Water Storage System Design for the Turkish Airlines’ New Istanbul Airport
When the government of Turkey made a bullish announcement to construct the world’s largest airport 30 kilometers away from the present Ataturk airport, it was incomprehensible for most of the architects and engineers to visualize such a mammoth project.
The project is spread over 167 hectares with capacity to accommodate 150 million passengers per year (PEX) and that presented several challenges to architects and engineers. Ghafari Associate LLC made big strides in designing complex airport systems around the world from Columbia, U.S., Saudi Arabia, Qatar, Abu Dhabi, China, Nicaragua, Philippines and India, but nobody had designed an airport of this magnitude and complexity.
Ghafari played a major role in the architectural and engineering design for numerous mission critical buildings with total area of 7.2 million square feet (700,000 square meters), including world’s largest catering facility with capacity to prepare 250,000 meals per day. Additional buildings included an air staff terminal, MRO, line maintenance hangar, cargo facility, operation center and three energy centers called TRIGEN plant to generate power, chilled water and steam/hot water simultaneously by utilizing natural gas.
This article will focus on the plumbing challenges and water storage facility design for the catering facility. It is a well-known fact that the Turkish Airlines’ sky meal is considered the best in the world and we had to be cognizant of the plumbing challenge to support their high standards.
We were mandated to provide storage for 1.5 days worth of water consumption. The facility was designed to prepare 250,000 meals per day for passengers and an additional 5,000 meals per day for the catering facility employees. The facility encompasses several specialty kitchens or spaces for preparation of multiple different cuisines such as Turkish, Arabic, Continental, Chinese, Indian, Italian, kosher, halal, bakery, breads, salads, desserts, business class meals, etc. The first challenge was to estimate peak water demand and the daily demand. Both numbers varied from country to country and from the facility operators to operators. For an example, water demand varied depending on different countries cuisines and from facility operators to operators. In general, water demand varied from 2.1 to 5.3 gallons (8 to 20 liters) per meal.
Another challenge was to estimate water consumption for 5,000 catering facility employees for bathing, toilet usage and other personal use. For example, India consumes 24 gallons (91 liters) per capita per day versus the U.S. consumption of 50 gallons (191 liters) per capita per day.
If we had based our calculations on 2.64 gallons (10 liters) per meal, then 660,000 gallons (2,500,000 liters) of water would be consumed per day. Conversely if we had based our calculations on 5.3 gallons (20 liters) per meal then the consumption would have jumped to 1,325,000 gallons (5 million liters) per day.
Engineers like to have a high safety margin so as to compensate for unknowns but the cost implications of over design can be colossal and at times not cost effective. A team of engineers from the Turkish Airlines and Ghafari visited existing facility as well as Singapore airlines’ state-of-art facility in Singapore to evaluate their operations.
We agreed that 2.64 gallons (10 liters) per meal water consumption was a prudent number. In addition, we built in the additional consumption of water for shower, toilet and other requirements.
Since we had to design multiple storage tanks for raw water, treated water and fire protection water for the initial consumption and back up, the space requirement for the storage tanks increased several folds. After several iterations, we ended up with total 494,400 ft3 (14,000 m3) water storage volume. The next challenge was to accommodate 494,400 ft3 (14,000 m3) of storage tanks and where. The decision was made to have a remotely located separate water tank building due to limited real estate availability in the proximity of the catering building.
We decided to go as deep as possible to reduce the water storage building’s footprint. After economic and hydraulic analysis, we concluded that the tanks should be 20 feet (6 meters) deep below the ground and 10 feet (3 meters) above the ground. Total 61,350 square feet (5,700 sq. meters) building with height of 30 feet (9 meters) was the conclusion. Structural engineers had to endure another challenge to design water tanks with strong foundations to bear water column load of 1,843 pounds-per-square-foot (9,000 kg per square meter) plus structural and miscellaneous weight. We accommodated 38 water pumps including fire pumps in addition to spaces required for softener plant, electrical room and telecom room in basement.
Water tank capacity was estimated based on the water demand for cooking, personal use, process demand, make-up water for cooling tower, drinking water demand and demands for washing and process application at the catering facility.
Raw water was provided by the utility company and we designed water storage with 1.5 days worth of water consumption. Raw water was treated in the softener plant to remove hardness. There were six main raw water tanks and they were further sub divided into 14 tanks because of structural constraints. Due to space constraints and site conditions, compartment sizes varied.
Treated water was stored in four smaller storage tanks with capacity equivalent to half of a day’s worth of consumption to meet peak and normal demands. Fire protection water storage capacity was designed as per NFPA with two tanks with 50 percent capacity to allow maintenance of one tank when required.
Each water tank’s outer walls and partition walls between tanks were designed as load bearing walls to avoid columns inside the tanks. Each tank was provided with stainless steel walking platform above water level to allow periodic inspection and maintenance and that created meters of unusable space.
Tank compartments were connected with each other with an equalizer line and isolation valve to maintain same water level in all tanks and also for easy maintenance. Manholes were provided at lower level in each tank for easy access.
Incoming water from utility company was connected to each compartment of the raw water and fire water tanks with motorized and manual isolation valves. These valves are operated when the sensor senses that the water level is below the set point.
Raw water is pumped through the treatment plant to the treated water storage tanks with the help of six 75 HP (55 Kw) transfer pumps with individual capacity of 770 GPM (175 M3 per hour) transfer pumps to fill the tanks.
The system is designed to supply treated water to various buildings by the booster pumps. Booster pumps are provided with variable frequency drive (VFD) and hydro pneumatic tank. Based on the economic analysis and energy consumption, two systems with separate pumping and piping were designed to supply water to the catering facility and other buildings. Four 100 HP (75 Kw) booster pumps with individual flow capacity of 700 GPM (160 M3 per hour) for the catering facility and four 60 HP (45 Kw) booster pumps with individual flow capacity of 440 GPM (100 M3 per hour) were provided to meet the peak demand.
All suction lines were provided with antivortex plate. Each tank has duplex water recirculation pumps of 50 GPM (11 m3-per-hour) capacity to recirculate water within tank with chlorine dosing system to maintain water quality. Recirculation system was designed to prevent water stagnation and development of foul odors.
Each tank has an overflow line and drainage line. Trenches with grate covers were provided in the floor and two sump pits were provided in the basement to pump out overflow water.
Duplex type sump pumps were provided in each sump pit with level sensors. Waste from sump pits was pumped outside the building and connected to external drainage lines.
Forced fresh air ventilation and exhaust was provided to remove foul odors inside each tank. Stainless steel ductwork were specified to eliminate corrosion problem. Heating and ventilation unit was provided with fresh air to maintain comfortable environment for the staff.
All equipment and accessories were connected with the BMS for remote monitoring. BMS has capability to monitor water usage, water level of each tank, status of pumps, status of motorized valves, failure of any system, sump pit water level, sump pumps run and on/off status, HVAC system status, pump room temperature, etc.
We learned that collaborative approach with client, facility operator and design engineers is the best solution to reach decisions on a large and complex project where risk of failure and over budget are significant. Designing large facilities in seismic zone creates another level of challenge. We exercised FMEA (Failure Mode and Effect Analysis) tool for vetting the design.
We like to express our gratitude to project managers, Dilek Yaicin and Selcuk Ibrahimglu, of Turkish airlines for tirelessly working with us to finish this monumental engineering task.
About the Author
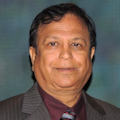
Nick Purohit
Managing Director, India Operations
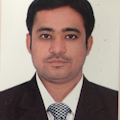