In the aerospace world, the procurement of spare parts is not always easy and is usually expensive, specially for older aircraft. At some point, when it comes to vintage aircraft, spare parts become rare, or in a worst case they simply don’t exist anymore. A historically significant airplane disappears from the airfield and becomes in a best case a static exhibit in the museum.
Today, with a help of reverse engineering technology the active life of such an airplane or entire fleet can be extended at a reasonable price. Till just a few years ago the failure of an engine part in a vintage airplane often was its death sentence. Now, there is a way to prevent the aircraft from being wrecked and to get them back in the air, even if there are no spare parts available.
Sheet metal parts are easier to reconstruct, engine parts, specially the big ones like engine blocks, are one much different and complicated story. This is mainly, because after decades casting molds don’t exist anymore - usually the original manufacturer went out of business decades ago and a new series production is not feasible for reasons of cost. In this case, reverse engineering technology now helps to reconstruct parts. This process, a kind of reverse development, makes it possible to re-create a product, which exactly equals to the original part, using an existing (broken) part, a piece of it, or a blueprint. In doing so, reverse engineering must go through a certain process, which includes the strategic consideration of how this "restoration" has to happen - where a specific succession of steps is required. This reverse engineering has many similarities with the design of a new part.
On the recovery path, from a damaged or no longer existing part, many problems have to be solved, because such a process is not simply a copy of the original part. Thanks to modern technologies such as 3-D scanning and new designer software, almost everything has become "doable," even with much better properties than the original part. An improvement of the properties is usually not (always) desired in aviation, since the parts have to meet the original certification standards. If reverse engineering is used for the commercial reproduction of parts, extensive industrial property rights and the corresponding licensing laws must also be observed. Parts for experimental aircraft can be reproduced in better quality, can be lighter, and cheaper, since less legal standards have to be met. A certified part has to be restored according to OEM (original equipment manufacturer) specification, as the original part was. It could, however, also be improved by more modern technology/materials, but this has to be coordinated by and with the OEM and the aviation authorities, otherwise the certification may be lost.
In a concrete example, which I have discovered at the last AirVenture in Oshkosh, I would like to explain the reverse engineering process. It does not always have to include all the described steps, some may be obsolete but sometimes some may be added - all taking into consideration the condition of the original part and many other factors.
This text describes a vintage airplane, engine crankcase remanufacturing done with a highly digitized process of two French companies: Ventana and Vintair. It is just an example of new remanufacturing possibilities offered by the use of modern technologies and proven traditional skills. It can be used in many fields like mechanical parts, sheet metal, molded parts, etc.
The Stampe SV4 - Biplane
The Stampe SV4 is a French aviation milestone. The Stampe, a little biplane, is part of the French aviation heritage and is famous for having been widely used in army and civilian flying schools in late '40s and early '50s. 850 airplanes were built in 1946-47, as an after WWII war reconstruction effort. The Stampe was built in France under license, and is based on a Belgian design from 1936. Those French-built Stampes were equipped with a 145-hp Renault, four-stroke, four-cylinder, in-line engine. Stampe features nice aerobatic capabilities, delightful handling, and has become a myth in the French aviation community. More than 200 airplanes exist, and are close to, or in a flying condition.
Material Fatigue As the Life Limiting Factor
One of the most challenging problems to keep Stampes flying is to find spare aluminum crankcases for the Renault engine. In-service crankcases and the (rare) spares available have logged a considerable number of hours. Like all light alloys parts they are subject to cyclic loads, where material fatigue is limiting their life. Many of the inspected crankcases show fatigue cracks.
Metallurgic analysis of the crankcases showed poor mechanical resistance, caused by impurities and internal corrosion, making the welding repair highly uncertain.
Because of this, some users started to consider moving to another engine. This is a very costly option, and no other engine allows it to stay close to the appearance and handling of the historic Stampe. For those reasons it was decided to remanufacture the crankcase using Ventana’s new techniques and Vintair reverse engineering skills offering a historically correct solution to the part shortage.
This is a typical example of the new technological possibilities when it comes to parts shortage. It can be applied to any other complex part and the whole process follows this path: Digitization of the used part. Using a high-precision optical scanner, the visible surfaces of the existing engine block are scanned creating a cluster of points with an accuracy of 0.02 millimeters creating a very good start point for a complete CAD reconstruction. To capture hidden details, like internal cavities or ducts, tomography technique may be used - the same complex and costly technique used in a medical scanner. Dedicated software allows to interpolate missing surfaces and to re-create the details using original design principles and geometry. When the highest accuracy is needed, a 3-D measuring robot is used for the most sensitive areas.
3-D Model, Manufacturing Drawings Setup
Most of the time, the data acquired by the optical scanner cannot directly be used, and a thorough functional analysis should be performed, what often requires careful measurement of adjacent parts.
A major step in this process is the CAD model creation - made by using all data acquired in the digitization step. This is a real reverse engineering step where dimensions and tolerances are determined to allow the final assembly, taking into the consideration the bearing alignment, gear axis matching, etc. If drawings/blueprints are available, they are extensively used, adding very useful information in this step.
In a case where no part and/or drawing is available, a new design can be established by using all available clues like pictures, location of adjacent parts, and a functional analysis.
Usually, when the material has been in service for many years, users will know the weakness areas of the part. While staying close to the original design, improvements may be introduced by using better suited alloys or slightly changing the geometry. This improvement process likely requires an approval of local aviation authorities through a minor or major modification request. As Stampe belongs to EASA Annex II aircraft list, this is done under French Civil Aviation Authority (DGAC) and the mod approval is granted to each aircraft owner.
Material Analysis
In a case that the original material/alloy is not known a mass spectrometer is used. Yield samples are used to assess the mechanical resistance of the material. This analysis allows the choice of the closest alloy amongst referenced materials. For this Renault crankcase - as no exact material match was found - a high resistance aluminum alloy was chosen. This is a real improvement but only possible for vintage planes under EASA Annex II rules, and under the owner's responsibility.
Casting, Solidification simulation
The crankcase is a sand casting made product, so the following step is performed in a foundry.
Once validated, the CAD model is directly used to design the casting mold. In this process a new, powerful simulation software is used, which helps to predict molten metal flow in the mold, temperature gradients, and metal shrinkage. This software simulation allows to avoid real-life testing and dramatically reduces development costs and time.
This phase ends with the sand casting mold design, where extensive foundry know-how is necessary, specially for parts with thin walls or relevant variations in wall thicknesses.
3D-Printing of Sand Cores
The cores which constitute the mold are then 3-D printed with a 3-D, S15 sand-printing machine. One layer of sand - to which one component of resin has been added - after another is applied on the "job box" a vertically moving table. Each layer of sand/resin is then polymerized - sprayed by a "print head" with a catalyst component - what binds the sand to a solid structure in a required form. When the mold is printed, the loose sand is mechanically removed (air blasted, brushed away) and the different cores are assembled to make the final mold.
When using this fully digital, tool-less process, complexity is no more a determining factor. Any part that has been cast can be easily remanufactured. 3-D sandprinting allows a high degree of shape complexity.
Sand printing of the mold sand cores is done by additive layer deposit (ALD) or additive manufacturing (AM).
It works like a big “jet printer” working over a vertically moving (lowering) flat “job box.”
A thin layer of sand is spread on the job box. This sand has been previously mixed with one of two components of a special resin. The sand thickness may vary between 0.3 and 1 mm, depending on the accuracy and speed required. The process is very sensitive to the type and amount of resin, and very specific sand is used, which allows a controlled thickness and granulometry of the layer. This is a part of Ventana’s know-how, that has been developed through a long setup, to be applied to widely used aeronautical magnesium- and aluminum-based alloys.
This thin layer of sand is then sprayed through the printer head with the resin’s catalyzer to build up the core where the sand has to remain solid. The loose sand that has not been sprayed is kept in place during the process to support the next layers and allows vaults and arch type geometries to be easily built.
This iterative cycle is repeated until the cores are completely built.
Multiple imbricated cores can be printed during the same session, permitting the full box volume use and reducing the (expensive) sand losses.
The mold is usually printed in several parts to allow a perfect cleaning (loose sand removal) and assembled before pouring.
After each layer has been sprayed where it should, the box lowers from the sand layer thickness, and another layer spreading and spraying cycle is started.
Printing can last from several hours to one or more days depending on multiple factors like requested accuracy, cores volume…
Casting
Molten metal is poured into the mold on a low-pressure casting station via a complex feeding network that ensures the integral filling of the mold.
Once the mold has cooled down, it is shot blasted to remove the remaining sand from the metal part, and the feeding network is cut away.
Inspection
The part then follows an inspection process that includes geometrical digital scanning, radiography, and other checks. The process is similar to the one used for the aeronautical jet engine parts manufactured by Ventana.
Heat Treatment
To achieve the desired mechanical properties, light alloys are heat treated.
Through this process, as it is identical to traditional foundry, the mechanical properties and resistance are strictly identical to a casting that would have been obtained through a traditional (not 3-D) pattern casting.
Testing
The complete range of NDT (nondestructive testing) is being implemented, from X-ray to automated (PT) fluorescent dye penetrating test - all according to the specifications of the contracting parties. Parts are checked for internal defects and correct metallurgical characteristics, all according to the specifications that are part of the reverse engineering process.
Mechanical Machining
The cast, if defect-free, is machined on a fully digital, high-precision CNC machines at the Ventana facility with the help of data obtained in the digitization process.
If needed, high-precision line boring can be performed, in its own traditional engine machining shop. This can include main and rod bearings boring.
Cost and Time
The costs and time exposure are difficult to determine in most reverse engineering processes because those figures are influenced by a number of factors. This depends on the nature and complexity of the part to be restored and the quality of delivered/available original part and blueprints. This process uses costly equipment and requires considerable design and engineering effort. While costs are higher than those of original parts that were manufactured in large quantities with a high degree of industrialization, they are much lower than traditional methods for limited quantities as no specific tooling is required, and real-life casting testing is dramatically reduced. Traditional, negative forms are not necessary, which for large series can cost several hundred thousand dollars.
Cost level is a function of part complexity and size, as well as NDT and alloy requirements.
Cost is split between nonrecurrent reverse engineering and mold design costs, and part production cost. Lead time can vary from several weeks for simple parts to three to six months when complex reverse engineering is required. The process is much faster than the standard foundry part development time.
AM of the above described part is somewhat simpler, since no molds are necessary. At a current cost of $500 per cubic inch of a structure, a motor housing would cost about $50,000 per piece - a lot more than this method.
So far, the absence of spare parts in the past meant a sure "death" of an aircraft. With this reverse engineering, the operational life of an historical aircraft can be extended at a manageable cost. Smaller batches are also affordable. The engine crankcase is only one example, a remanufactured brake drum of the Junkers JU52, which was no longer available, was also built. There are just few similar projects in the USA and Europe that will lead to whole aircraft in the next two to three years with the use of reverse engineering. For example, Replic Air from Toulouse, France is building the Le Dewoitine D551, an aircraft destroyed before its maiden flight in WW2 (www.replicair.fr). In such large-scale projects, the fuselages and wings are "relatively" easy to manufacture because they are made of wood or sheet metal. However, problems arise with nonexisting undercarriage and engine components. Here reverse engineering can make a project feasible.
Currently the maximum possible size of a part depends on the dimension of a job box what still limits the production of some parts. Software and testing techniques must also be further developed and adapted. So far, there are only a handful of companies in the world that use this method. With the fast growth of 3-D printing technologies and machines, this will hopefully change soon. For more information visit www.vintair.com or www.ventana-group.eu.
Ventana and Vintair
A Renault engine housing is being remanufactured for a Stampe SV4 airplane, with French certification. In this example, the French companies Ventana and Vintair cooperate and manufacture a crankcase using a highly digitalized process.
Ventana is a major French aerospace subcontractor. With its four foundry facilities, high precision machining and sheet metal capabilities, Ventana covers many of the required fields and competences when it comes to remanufacturing. Ventana customers are located worldwide, including Airbus, Rolls Royce, and Pratt & Whitney.
Vintair is a small company dedicated to vintage and collection aircraft. It specializes in restoration of vintage engines and has developed reverse engineering skills useful in that field.
About the Author
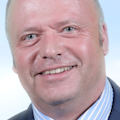
Marino Boric
Marino Boric graduated with a university degree as an aeronautic engineer, and acquired degrees in business development/trade and commerce and in journalism. He is a civil and military pilot and has built experimental aircraft. As a journalist, he specializes in aviation and propulsion and travels worldwide, flight-testing UL, LSA, Experimental, and certified aircraft. He is writing for U.S., European, and Chinese media companies.