Once upon a time in the 1800s, there was a scrupulous group of scientists participating in experimentation with electricity and magnetism without being classified as alchemists or wizards. (Sorry, J.K. Rowling.)
In 1802, Gian Domenico Romagnosi suggested the relationship between electric current and magnetism, but his reports went unnoticed. Less than 20 years later, Hans Christian Orsted performed a widely known experiment on man-made electric current and magnetism where he demonstrated that a wire carrying a current could deflect a magnetized compass needle. Then there was Nikola Tesla, who determined the feasibility of wireless communications and developed means to reliably produce radio frequency currents. Tesla’s U.S. patent classified him as the “inventor of radio.”
Guglielmo Marconi, who is also often classified as the “father of radio,” eventually equipped ships with life-saving wireless radios and conducted a reported transatlantic radio communications experiment in 1901. He then established the first commercial transatlantic radio service in 1907, a mere 20 years prior to Charles Lindbergh’s oceanic crossing.
Radio waves
Speaking of oceanic, sea currents — like radio signals — travel via waves. Just like their watery counterparts, radio waves are made up of peaks and valleys. Radio waves are usually produced by electric current alternating at radio frequency, flowing in a special purpose conductor called an antenna. Antenna dimensions must be specific to wavelength and tuned to work efficiently in a selected frequency range. Very long radio waves are not practical for aviation use because of the enormous antennas needed to produce them. Radio waves do occur naturally as a result of cosmic phenomena in deep space. In fact, any kind of reciprocating motion of electric charges or magnets can produce radio waves if the interaction is fast enough.
Propagation is a term that describes the travel of electromagnetic waves and consists of three modes. The first is a straight line travel, the manner that radio waves travel through deep space. The second way is to hug the surface of the earth and consists mainly of radio waves operating at very low frequency. Skip is the third mode –- as the name implies, it involves bouncing radio waves between the surface of the earth and the ionosphere.
Frequencies between 3 Megacycles or MegaHertz (MHz) and 30 MHz are most reliable for this kind of propagation and are known as high frequency (HF) waves.
Most aircraft communication today will involve the use of “line of sight” transmission and operate in the very high frequency (VHF) spectrum. This was not always the case in aviation. When dedicated communications radios took flight they were initially of the tried and true HF variety.
Radio waves travel in straight lines (except at very low frequencies) and the earth is curved, so we should not be able to communicate with anything we can’t see. In the Titanic era, ships used very low frequencies (500 KHz) to take advantage of a bending effect called refraction, but even then the range was only about 1,000 miles. (This is called the Ground Wave mode.) Above about 3 MHz, this effect is less pronounced. At the time it was quite unexpected that these shorter wavelengths could travel across the Atlantic Ocean or worldwide. The phenomena became known as Short Wave Radio.
Soon it was realized that the reflection of the waves from the ionosphere (Sky Wave Mode) could, under certain conditions, allow reliable long-range communication. This was of great interest to maritime operators and is still in use with ships today. Marine operators could send telegrams by Morse code, tele-printer over radio systems (TOR), or phone calls by single side band (SSB), especially to set up radio stations back in their home countries.
Current technology can even make HF radio an efficient means of sending and receiving email.
Why is the ionosphere important?
The ionosphere is a region of electrically charged particles or gases in the earth’s atmosphere, extending from approximately 30 to 375 miles above the earth’s surface.
Ionization, the process in which electrons are stripped from atoms and produce electrically charged particles, results from solar radiation. When the ionosphere becomes heavily ionized, the gases may even glow and be visible. This phenomenon is known as Northern and Southern Lights. Why is the ionosphere important in HF radio? This blanket of gases is like nature’s satellite and makes most Beyond Line Of Sight (BLOS) radio communications possible. When radio waves strike these ionized layers, some are completely absorbed. Depending on frequency, others are refracted so that they return to the earth while still others pass through the ionosphere into outer space. Absorption tends to be greater at lower frequencies and increases proportionally with the degree of ionization.
The angle at which sky waves enter the ionosphere is known as the incident angle. This is determined by wavelength and the type of transmitting antenna. Like a billiard ball bouncing off a rail, a radio wave reflects from the ionosphere at the same angle at which it hits. Incident angle is an important factor in determining communications range; to reach a station that is relatively far away, the incident angle has to be relatively large. In order to communicate with a nearby station the incident angle should be relatively small. If the incident angle is nearly vertical, it may pass through the ionosphere without being refracted back to earth. If the angle is too great, the waves will be absorbed by the lower layers before reaching the more densely ionized upper layers. Therefore, the incident angle must be sufficient to bring the radio wave back to earth, yet not so great that it will lead to absorption.
Within the ionosphere, there are four layers of varying ionization. Since ionization is caused by solar radiation, the higher layers of the ionosphere tend to have a greater density, while the lower layers experience less ionization. Of these layers, the first, discovered in the early 1920s, was designated E for electric waves. Layers D and F were discovered later.
Additional theories related to the ionosphere were explored through the 1930s and 1940s, such as sporadic E and aurora. The D layer is the lowest region affecting HF radio waves. Ionized only during the day, the D layer reaches maximum ionization when the sun is at its zenith and dissipates quickly toward sunset.
The E layer reaches maximum ionization at noon. It begins dissipating toward sunset and reaches minimum activity at midnight. Irregular cloud-like formations of ionized gases occasionally occur in the E layer. These regions, known as sporadic E, can support propagation of sky waves at the upper end of the HF band and beyond.
The most heavily ionized region of the ionosphere, and therefore the most important for long-haul communications, is the F layer. At this altitude, the air is thin enough that the ions and electrons recombine very slowly, so the layer retains its ionized properties even after sunset. In the daytime, the F layer consists of two distinct layers, F1 and F2. The F1 layer, which exists only in the daytime and is negligible in winter, is not important to HF communications. The F2 layer reaches maximum ionization at noon and remains charged at night, gradually decreasing to a minimum just before sunrise.
During the day, sky wave reflection from the F2 layer requires wavelengths short enough to penetrate the ionized D and E layers, but not so short as to pass through the F layer. Generally, frequencies from 10 to 20 MHz will accomplish this, but the same frequencies used at night would penetrate the F layer and pass into outer space. The most effective frequencies for long-haul nighttime communications are normally between 3 and 8 MHz.
As frequency is reduced, the amount of absorption of the signal by the D layer increases. Eventually, the signal is completely absorbed by the ionosphere. The frequency at which this occurs is called the Lowest Usable Frequency (LUF). The “window” of usable frequencies lies between Maximum Usable Frequency (MUF) and LUF.
The Frequency of Optimum Transmission (FOT) is nominally 85 percent of the MUF. Generally, the FOT is lower at night and higher during the day.
In addition to frequency, the route the radio signal travels must also be considered in optimizing communications. A received signal may be comprised of components arriving via several routes, including one or more sky wave paths and a ground wave path. The arrival times of these components differ because of differences in path length; the range of time differences is the multi-path spread. The effects of this can be minimized by selecting a frequency as close as possible to the MUF.
Since many of the variables affecting propagation follow repetitive cycles and can be predicted, techniques for effectively determining FOT have been developed.
A number of propagation prediction computer programs are available. One such widely used and effective program is Ionospheric Communications Analysis and Prediction (IONCAP), which predicts system performance at given times of day as a function of frequency for a given HF path and a specified complement of equipment.
A closer look at the components
The components in an HF radio system fall into three groups: transmitters, receivers, and antennas. In many modern radio sets, the transmitter and receiver are contained in a single unit called a transceiver.
Most aviation applications will require a flight deck located control panel. This is where the system can be switched on and tuned. Other features include squelch that allows noise reduction and a clarifier that modifies the frequency oscillator within the receiver to be fine-tuned to improve signal quality. Many units include switches to allow storing frequencies and a mode select for side band operation. This feature gives an increase in range but distorts the voice quality. Newer controllers may also include a selector for messages.
A receiver/exciter is the device that will demodulate the signal captured by the antenna and supply the audio portion to the flight crew. One common option is selective calling (or SELCAL). This feature will bring the crew’s attention to calls to their specific aircraft and is accomplished with a recognition code implanted on the transmitted signal. Once a SELCAL-equipped signal reaches the system, it is decoded and either an aural or visual alert is provided to the flight crew.
Transmitters vary in construction, though they all contain a means of producing a carrier wave as well as a method of imposing the information to be transmitted on the carrier. Power amplifiers, either internal or external, will then adjust the final output to achieve an appropriate broadcast range. The exciter synthesizes a carrier, which is combined with a lower frequency signal derived from a source of information such as a microphone. The resulting signal is converted to the frequency that is to be transmitted.
Tuning or frequency selection is achieved in modern transceivers by using electronic micro-circuits. Some equipment uses crystal control for achieving desired frequency. The power amplifier boosts the output power of the signal to the desired wattage for transmission before sending it through a cable to the antenna. In addition, the transmitter may contain filters that are used to clean the output.
Once the signal reaches the antenna it is propagated into the atmosphere. HF communications systems on aircraft employ several types of antenna including “Short Wire, Long Wire, V and Shunt.”
The wing type “V” is probably the most effective for slow to moderate speed aircraft. With a length usually between 9.2 to 13.7 meters, the radiation pattern of the transmitted signal is mostly omni-directional and exhibits efficiency at all HF band radio frequencies. Disadvantages include being a physical obstacle and high drag, especially on low wing aircraft.
A shunt antenna built into the leading edge of the vertical stabilizer is most common on high performance aircraft. There is no additional drag and the radiation pattern is practically omni-directional. Efficiency is generally good, but can deteriorate as a result of poor electrical bonding.
Tuning the antenna will allow greater efficiency through the operating frequency spectrum. The wavelength of a radiated signal is determined by the frequency and speed of transmission. The speed is a constant at 300,000,000 meters (186,000 miles) per second, which is also the speed of light. Dividing the speed by the frequency will result in the wavelength. As an example, a 10 MHz transmission will have a wavelength of 30 meters.
In most modern day installations, the antenna is a fixed length. The only way to compensate it for frequency variations is through tuning. The variables here are inductance (some antennas are enhanced by adding coils of wire) or capacitance. Some HF system manufacturers install a variable capacitor in line with the antenna and will alter this value allowing the antenna to operate at acceptable levels throughout the intended frequency spectrum. Other installations will accomplish antenna tuning in the antenna coupler.
HF radio has been the means of communication of choice for mariners. The onset of affordable satellite communication has impacted the use of HF and many aeronautical HF radio service providers have switched off their systems. However, the capability of HF to transmit data far exceeds the current capability of the Iridium satellite telephone network. Equipment pricing of HF radio is far below that required to utilize the Inmarsat Satcom constellation.
In recent years, this data capability has breathed new life into an age-old technology. With flight deck available data being the main focus of enhanced air traffic management and communications, it appears HF radio systems will be with us for many years to come.
The moral of this story is obvious: it is better to transmit than to receive (sometimes).
Jim Sparks has been in aviation for 30 years and is a licensed A&P. Currently when not writing for AMT, he is the manager of aviation maintenance for a private company with a fleet including light single engine aircraft, helicopters, and several types of business jets.
About the Author
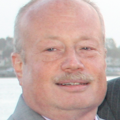
Jim Sparks
Jim Sparks has been maintaining aircraft for almost 40 years with the majority of the time involving Business Aviation activities. Jim’s endeavors have placed him on six of the seven continents contending with numerous situations from routine flight dispatch to critical AOGs. His career includes maintainer, avionics/electrician, educator, tech rep, and director of aircraft maintenance. In addition to other activities he is engaged with ASTM assisting in the global development of criteria defining the Next Tech for NEXTGEN. You can reach him at [email protected].